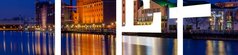
Research in Collaborative Research Center 1242 “Non-Equilibrium Dynamics of Condensed Matter in the Time Domain“
With sufficiently short-term external stimuli such as light flashes, impulsive pressure changes, electrical voltage surges or particle impact, non-equilibrium states can be prepared in condensed matter, which cannot be achieved with a static energy supply. The strong excitations of the electrons and lattice oscillations in the solid state change very dynamically in time and space, with characteristic time and length scales up to the femtosecond and nanometer ranges being of particular interest to the members of CRC 1242. They can only get there with the most modern detection methods, for example in optics, electron spectroscopy and electron or X-ray diffraction. These allow processes to be tracked in real time on the microscopic time scales on which atoms and electrons move in matter – comparable to films recorded with high-speed cameras but several orders of magnitude faster.
Several projects are presented below.
Ultrashort and Terahertz physics
In 2017 to 2018, the Turchinovich research group was involved in the projects focusing on THz physics of graphene and THz spin electronics. One of the most important outcomes was demonstration of extremely efficient terahertz high-harmonic generation in graphene.
If electrons in graphene are excited by a multicycle quasi-monochromatic THz wave, the oscillation of the graphene conductivity resulting from the heating and cooling dynamics of the electrons leads to a highly nonlinear electrical current in the graphene, which leads to electromagnetic radiation at higher harmonics than the driving THz frequency. The members of the Turchinovich group discovered that graphene has nonlinear optical THz coefficients that are many orders of magnitude higher than those of other electronic materials, which may make graphene the most nonlinear material known to date.
Time-resolved electron microscopy
In the field of time-resolved electron microscopy on electron density waves, the work of the Horn-von Hoegen and Meyer zu Heringdorf research groups concentrated on properties of surface plasmon polaritons (SPPs), longitudinal waves at an interface that are capable of propagating in the electron system of selected noble metals (e.g. gold, silver). Surface structuring with focused ion beams permits targeted control of the optical excitation of SPPs , making it possible to influence the form of the electron density wavefronts more precisely. In time-resolved photoemission microscopy, the waves can be “filmed” in super-slow motion as they move over the surface virtually at the speed of light in a vacuum.
A highlight of this research is the work being conducted on two-dimensional plasmonic singularities generated by spiral excitation structures. Like in a spiral staircase, where the riser height at its centre is indeterminate (the reason there always has to be a hole or a post in the middle of a spiral staircase), the phase of the SPP wavefront at the centre of the excitation structure is also indeterminate. A singularity occurs, and the centre of the structure is shielded for topological reasons at all times from the SPP.
This study is the result of close cooperation with research groups from Stuttgart, Kaiserslautern and Haifa (Israel). Its publication is the basis for further studies, which also benefited in 2018 from a visit by Prof. Tim Davis from the University of Melbourne (Australia).
Nanoscale oxides for energy conversion
A focus of research in Prof. Rossitza Pentcheva’s group is on thermoelectric materials for converting waste heat into electrical energy. The group’s latest studies show that the necessary n- and p-type systems can be produced from the same combination of materials by varying the order of the layers at the so-called polar interfaces in a specific way. Reduced dimensions are another possibility for improving thermoelectric properties: in particular, superlattices comprising a monolayer of the correlated metal lanthanum nickelate and of the insulator lanthanum aluminate show a significantly higher Seebeck coefficient under tension.
An extremely topical and simultaneously challenging issue is modelling the time evolution of laser-induced electronic excitations in bulk materials and at interfaces, which is the subject of a project in Collaborative Research Centre 1242 in collaboration with colleagues from Experimental Physics.
Two-dimensional semiconductors
The discovery of graphene has inspired many researchers to search for other comparable materials that consist of only a few atomic layers. They are looking in particular for two-dimensional atomic layers that, unlike graphene, have the electrical properties of a semiconductor. Chemical compounds of the elements gallium or indium with sulphur or selenium have precisely these properties and can, like graphene, be fabricated by “de-layering” a multilayer crystal. This makes it possible to also compose artificial layered structures of several different materials, which can be under mechanical tension. Members of the Kratzer research group have computed the cohesion of such stacks of layers and explored the changed electronic properties compared to those of the individual materials. Future research will look at possible applications for these types of materials in photovoltaics.
2D materials
The Schleberger research group is concerned with the fabrication and study of 2D materials. 2D materials are ultrathin (usually between one and three atomic layers) yet surprisingly stable. This combination makes them interesting for many applications. For example, they can be made into filters, membranes for sequencing, or transparent electrodes. Methods are currently being developed for CRC 1242 to fabricate special large-scale self-supporting carbon membranes. These graphene membranes withstand bombardment with ions but emit electrons in the process. A special camera makes time-resolved tracking of these electrons possible, which means that they act as a measure of time for the length of the ionic pulse. This is an important component of the ultrashort-pulse ion source that the Wucher, Sokolowski-Tinten and Schleberger research groups are jointly developing in CRC 1242.
Tracking charges
The smallest electrical charge is the elementary charge e0. All observable charges are integer multiples of e0. An electron carries a negative elementary charge, for example, and a proton a positive one. The ability to measure the smallest changes in a charge is extremely important for understanding photo effects, chemical reactions, nanoscale mechanical impact or for investigating electrostatically charged dust particles and droplets as they occur in many technical processes. A current area of research in Prof. Möller and Prof. Nienhaus’s group concerns fast measurement of extremely small charge currents. Using a cooled junction field effect transistor (JFET), it was possible to detect currents under one elementary charge per second (<10–19 A), which is significantly better than any commercial electroscope. The method is currently being used in the lab to precisely measure charge transfer processes using the example of charged spheres impacting on metal plates. If, as shown in the figure, a charged sphere drops onto the plate of a capacitor, the sphere bounces several times until it loses its kinetic energy. The charge can be transferred between the sphere and plate each time they contact. This becomes apparent if the induced and transmitted charge is measured in time with the JFET. The figure shows an example of a 1-mm-diameter steel sphere hitting a copper plate. From the parabolic curves it is possible to study quantitatively details of the charge and energy transfer. Initially, the sphere drops onto the plate with a positive charge of 47 fC (approx. 290000 e0). The first and second time the sphere contacts with the plate, its polarity is reversed, which leads to inversion of the parabola. Every other time the sphere impacts, the amount of charge on it changes, but not the polarity. The background to these measurements is current astrophysics research, which is seeking to explain the role collisions between charged dust particles or chondrules in protoplanetary disks play in planetary evolution.